How to engineer a renewable deuterium–helium-3 fusion fuel cycle
Lily Hale, Fuel Cycle Team Manager
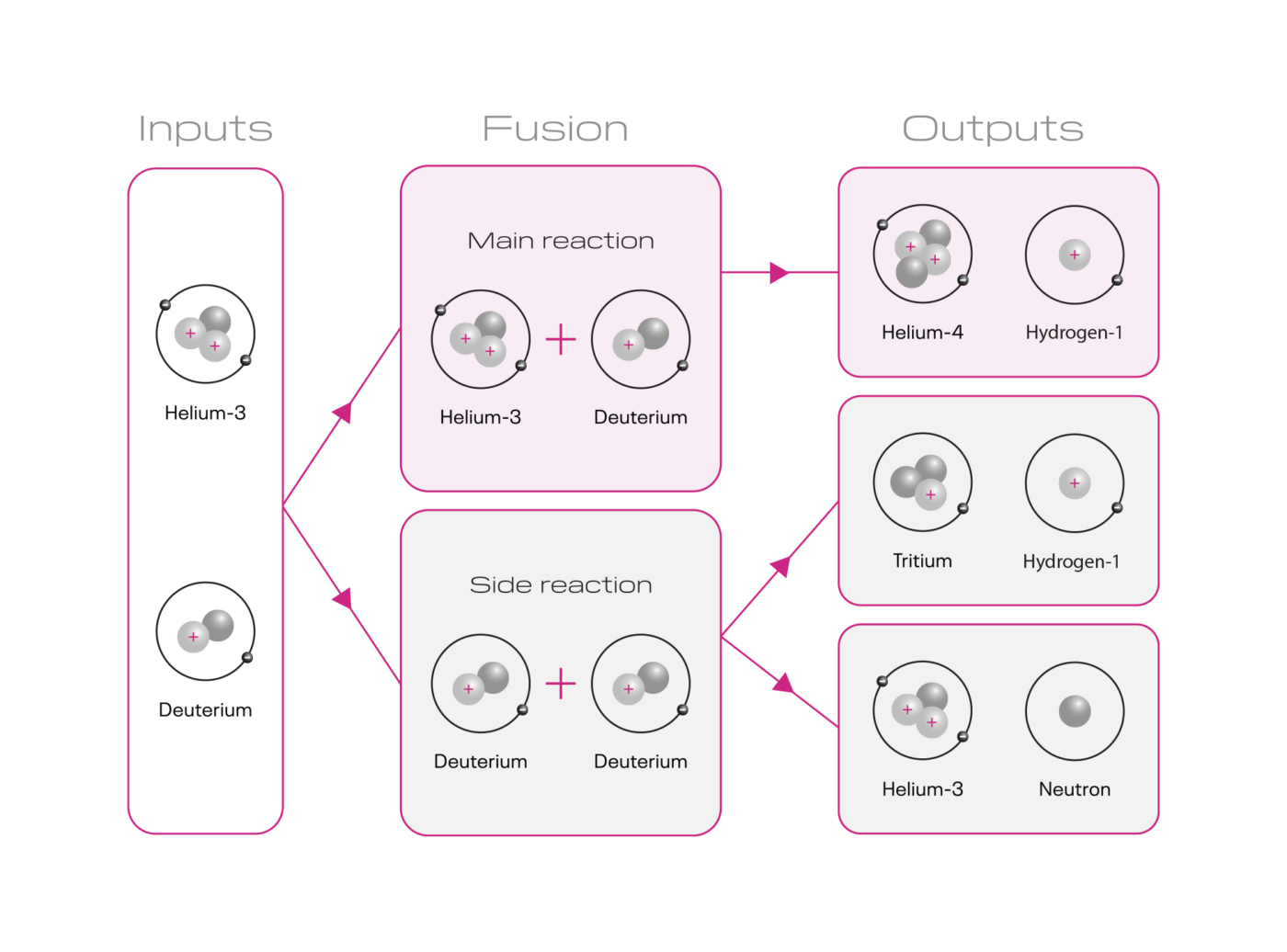
Helion’s fuel of choice is deuterium–helium-3 (D–3He). This combination offers several key advantages; chief among them is the production of charged particles that we can directly convert to electricity, leveraging much higher efficiencies than that of thermal conversion. However, helium-3 is exceedingly rare on Earth with no known natural source to supply the quantities necessary for a fleet of fusion machines. Without a sustainable helium-3 supply, scaling D–3He fusion power to commercial levels is impractical. While some suggest mining lunar regolith for helium-3, these prospects remain economically and technologically speculative.
To create electricity from fusion using a D–³He fuel cycle, we must engineer a machine that can both operate with and abundantly generate fuels for sustained use. A promising solution naturally emerges in the form of a closed-loop D–³He fuel cycle.
D–D reactions create helium-3
While our machine primarily runs D–3He reactions for power production, D–D fusion is a co-reaction that contributes to helium-3 production in both immediate and delayed forms. Deuterium atoms fuse with each other to produce helium-3 through two pathways. Half of the time D–D fusion directly yields helium-3. The remaining half produces tritium, which serves as a stored resource that transforms at a rate of 5.5% per year into helium-3 through natural radioactive decay with a 12.3-year half-life.
This two-path system works as a fuel supply investment plan—part gives you helium-3 returns immediately, while the rest grows for the future. In fact, this fuel cycle system strengthens itself over time; the longer a machine runs, the more self-sufficient it becomes in producing helium-3. This self-reinforcing nature is what gives me confidence that we can produce sufficient quantities of helium-3 to operate our advanced fuel cycle.
Engineering the fuel cycle
A fusion fuel cycle is the pathway for removing, processing, and reinjecting fuel to the machine in a safe, routine manner. What makes this engineering challenge unique is the need to harmonize processes across vastly different timescales. Plasma pulses occur in microseconds, fuel separation takes minutes to hours, while tritium decays into helium-3 over years.
Our fuel systems must bridge these timescales by drawing on proven techniques from nuclear and chemical engineering. After each pulse, tritium and byproducts must be separated from helium-3, using divertors, membranes, and cryogenic pumping. Tritium is collected, purified through established isotope separation processes, and then stored for future helium-3 production.
For tritium storage, we utilize getter materials—specialized metal alloys that bind tritium in solid form while allowing controlled release of helium-3 as it forms. Tritium management is the bridge between today’s operations and tomorrow’s fuel supply. Tracking, guided by ALARA (As Low As Reasonably Achievable) principles, serves as both a safety compass for radiation protection and a fuel ledger for helium-3 management. Real-time continuous analysis throughout the cycle optimizes our fuel supply.
Balancing fuel consumption and production
A sustainable cycle hinges on the balance of two complementary processes: D-³He fusion (which generates more power and consumes helium-3) and D–D fusion (which produces helium-3 and yields less power). The range in degree of contributing reactions allows for a flexible operational approach.
This means we could begin with lower concentrations of helium-3, gradually increasing D–³He reactions as more becomes available. Over time, the system may reach a steady state where helium-3 production is balanced with power generation, enabling sustained operation. With careful control of this equilibrium, a closed-loop system requiring only deuterium input is achievable.
Demonstrating the complete fuel cycle
Polaris, our seventh-generation fusion machine, represents a crucial transition from concept to demonstration. By testing all aspects of the closed-loop fuel cycle with multiple fuel combinations, this system validates both individual components and their collective operation—a necessary step in moving from promising technology to practical energy solution.
Supporting this effort, our new fuel processing laboratory prototypes and refines technologies necessary for fuel recycling, isotope separation, and tritium handling. It enables us to establish procedures, train authorized users of fusion fuels, test and validate platforms for tritium processing and storage in a safe environment, and optimize analytical tools for exhaust and fuel purity assessments. Tackling research and development of these systems now will mitigate technical risks surrounding the fuel cycle before our first power plant begins operating.
A self-sustaining solution
A renewable deuterium–helium-3 fusion fuel cycle holds significant promise for addressing global energy needs and mitigating the impacts of climate change. By producing helium-3 through D–D reactions and tritium decay, the approach eliminates dependence on scarce external sources while maintaining the advantages of D–3He fusion.